Open Access Journal of Biomedical Engineering and Biosciences
Abstract
Factors influencing the cancer therapy efficiency in both
photothermal therapy (PTT) and photodynamic therapy (PDT) using nanogold
particles and photosensitizers, respectively, are analyzed. In PTT,
heat diffusion kinetics is used to calculate the temperature increase
resulted from the nanogold absorption of light energy, whereas
photochemical kinetics is used to find the efficacy of PDT, or the
generation rate of reactive oxygen species. Efficacy of cancer therapy
may be enhanced by combining PTT and PDT either activated by one light
or two lights. For maximum PTT/PDT synergistic efficacy, the
concentration of photosensitizers and nanogold required optimization,
besides the wavelength of the light matching the absorption peak of PS
and nanogold, and the sequential order of PTT and PDT process. External
supply of either photosensitizers or oxygen concentration will
significantly improve the anti-cancer efficacy via type-II PDT.
Optimization is required for maximum synergic efficacy.
Keywords:Photothermal Therapy; Optimal; Synergistic effect; Modeling; Heat diffusion; Photochemical kinetics
Abbrevations: PTT: Photothermal Therapy;
PDT: Photodynamic Therapy; IND: Investigational New Drug; NRI:
Near-Infrared; GNR: Gold Nanorod; PS: Photosensitizers; MB: Methylene
Blue
Introduction
Various methods/technologies have been investigated for the
improvement of phototherapy of cancers, including nanomedicine,
nano-particles and synergic method combining photothermal therapy (PTT)
and photodynamic therapy (PDT) using nanogold particles and
photosensitizers, respectively [1-10]. Cancer or tumor cells death may
be caused by photothermal ablation, mechanical damage, and increase in
the localized drug concentration. Gold nanoparticles are promising
agents for cancer therapy, drug carriers, photo-thermal agents and
contrast agents. The U.S. FDA has approved numerous Investigational New
Drug (IND) applications for nano-formulations, enabling clinical trials
for breast, gynecological, solid tumor, lung, mesenchymal tissue,
lymphoma, central nervous system and genito-urinary cancer treatments.
Comparing to the visible light, near-infrared (NIR) light offers the
advantages of larger absorption and scattering cross sections and much
deeper penetration depth in tissues [5-8].
The red-shift of the absorption peak in nanorods is governed by the
aspect ratio (defined by as the ratio of the length to the
crosssectional diameter), whereas it is governed by the shell thickness
in nanoshells [9].
Recent studies have shown that gold nanorods conjugated to antibodies
[11-13] could be used for selective and efficient photothermal therapy.
Lin et al. [4] proposed the use of a diode laser system having multiple
wavelengths for more efficient treatment of cancer tumor. Overheating
on the surface area of targeted tissues is always an issue to be
overcome. In addition, the distribution of the gold nanorod (GNR) aspect
ratios and their concentrations inside the cancer tissues or tumors are
also difficult to be controlled for perfectly matching the laser peak
absorption. To overcome the penetration issue, Lin et al proposed the
use of a train-pulse to increase the volume temperature increase [4]
which is particularly useful to larger volume tumors, unless an
inserting fiber is used
to deliver the laser energy. New synergistic treatment modalities
combining PDT with PTT could overcome current limitations of PDT, thus
achieving enhanced anticancer efficacy. To promote the tumor
accumulation of photosensitizers (PS) and to generate heat for
synergistic PDT/PTT [10], surface conjugation of PS on nanoparticles has
been proposed, which however, has limitations including relatively low
loading capacity and the possible leakage of PSs coupled on nanoparticle
surfaces during their circulation in biological systems.
In this study, we will review the factors influencing the cancer
therapy efficiency in both PTT and PDT using nanogold particles and
photosensitizers, respectively. In PTT, heat diffusion kinetics is used
to calculate the temperature increase resulted from the nanogold
absorption of light energy, whereas photochemical kinetics is used to
find the efficacy of PDT, or the generation rate of reactive oxygen
species. Besides a review, the measured data of synergistic PDT/PTT [10]
will be discussed. We will also present new optimal parameters for
maximum PDT efficacy.
Methods and Discussions
The Modeling System
Figure 1: Combined PTT and PTT processes using various lights
having wavelength from UV to IR with associate nanogold shapes and
photosensitizer [2].
As shown in Figure 1, the tumor cells killing efficiency may be
enhanced by combining PTT and PDT using two light sources (either lasers
or LED sources), in which the treated tumor tissue is injected by both
nanogold solution and photosensitizers [2]. Depending on the types of
photosensitizers and the shapes of the nanogold, the light wavelengths
matching the absorption may vary from UV, visible to near IR (NIR). For
examples, nanosphere absorbs visible light (at 480-680 nm), nanotubes
(700-900 nm), nanorod (700-2500 nm), and nanoshell (480-810 nm) [1]. As
shown in Figure 1, the combined PTT and PTT processes using various
lights having wavelength from UV to IR with associate nanogold shapes
and photosensitizer. Photosensitizer riboflavin (B2), 5-ALA, methylene
blue (MB) and indocyanine green absorb, respectively, light at
wavelength of (365, 430nm), (530-670nm), (780-850nm), as shown by Figure
2 [1,10]. Therefore, a combined dual-function of PTT/PDT can be
performed by: (i) an NIR light at NIR absorbed by gold nanorod and
indocyanine green; or a visible light absorbed by gold nanosphere and
5-ALA; (b) two different lights having wavelength at NIR (for PTT) and
UV to visible light (for PDT). For the case of one light for both PTT
and PDT the simultaneously interacting with the nanogold and the
photosensitizer is much more complex that that of the case of two
different lights which can be treated independently. We will start with
the simpler case, where PTT and PDT will be modelled by the heat
diffusion equation and the kinetic equation, respectively, as follows.
Figure 2: Measured dT profiles for a fixed light fluence F=2.8 (W/cm
2) with A=3.0 (solid curves) and 1.0 cm
-1 (dash curve) [4].
The Temperature Increase in PTT
The temperature profile of the laser irradiated GNR solutions may be
solved numerically by a heat diffusion equation given by [4]
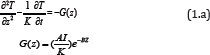
where z is the laser propagation direction along the depth of the
GNRs solution, k and K are, respectively, the thermal conductivity and
diffusivity of the solution, I is the laser intensity (or power
density), B is the extinction coefficient, which can be expressed by
B = [A(A + 2s)]
1/2,
where A and s are the absorption and scattering coefficient. In this
study we will assume that the scattering is much smaller than the
absorption, and B=A. We note that A is proportional to the product of
the extinction coefficient and concentration of the GNRs.
Optimal conditions in PTT
The light source terms G(z) have an optimal value. By taking
dG /
dA (A = A *) = 0 ,one obtains the maximal
G* =
I / (
zK) under the optimal condition
A* =
1/ Z. The maximal G* is resulted from the competing function of A and
exp(-Bz). The optimal A* also indicates that there is an optimal GNR
concentration, C*, since A is proportional to C. Lin et al proposed a
novel pulsed-train method (PTM) to overcome the surface overheating
issue (in conventional CW mode) and improve the volume temperature
increase which is required for large volume tumors [4]. In our
laboratory test, we demonstrated that the temperature increase profiles
(dT). Surface- dT about 100C by the pulsed train on-off technique such
that the volume-dT (at z=5.0 mm) reaches about 80C, which cannot be
achieved in CW mode operation without overheating the solution surface.
In addition, higher laser intensity shows faster surface-dT rising
profile and higher volume-dT inside the solution (Figure 2).
We note that the PTM along cannot increase the volume-dT to the
desired value. One shall also require an optimal A value (say about
1.0cm
-1 to 1.5cm
-1) which may be controlled either
by the GNRs concentration or by using specific off-resonance laser
wavelengths. Moreover, the A-value cannot be too small (say <0.5 cm
-1)
which will require a longer time needed for a surface-dT reaching 10
0C. The novel features demonstrated in the above described also imply
that cancer tumor having a dimension of 10x10mm can be treated using the
PTM, but not by the conventional single pulse method Figure 2. Shows
the measured surface and volume dT profiles for a fixed light fluence
F=2.8 (W/cm
2) with A=3.0 (solid curves) and 1.0cm
-1 (dash curve), where the smaller A has higher volume temperature [4].
The in vivo situation in animal and/or human cancer therapy will be
much more complex than the in vitro, simplified conditions described in
above cancer cell model. These complexities shall include the
non-uniform GNRs concentration in the tumor, the multi-layer
normal-cancer tissue medium with multiple thermal parameters, and the
blood flowing of the laser-targeted areas. In addition, the design of
multiple-wavelengths laser system shall partially overcome the issues of
GNRs non-uniform and multiple thermal medium for a
3-dimensional-therapy, in which various absorption penetration depths
are available via the fiber-coupled multiple-wavelength laser
simultaneously targeting the cancer tumors, The novel PTM and the laser
system with auto-temperature control may provide useful tool for animal
studies, where a fast temperature response time given by an IR camera
may be integrated for real-time surface temperature monitoring.
The combined PTT/PDT efficacy
PDT process utilizes reactive oxygen species (ROS) generated through
the reaction between photosensitizer (PS) and oxygen presented in
tissues upon the irradiation of light to achieve effective treatment.
The ROS is generated under a so-called type- II photochemical reaction
which requires oxygen. In comparison, type-I process does not need
oxygen and the triplet PS state can interact directly with the target
for anti-cancer.
Using the same light for both the PS and nanogold interaction is much
more complex than when two light with different wavelengths are
absorbed respectively by the PS and nanogold, in which the PTT and PDT
can be treated independently. Combining PTT and PDT using a single
light, the kinetic equations of the light intensity I(z,t), the PS
concentration C(z,t) and oxygen concentration, [
3O
2] =Y, are given by [3]
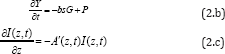
Where b=aqI (z, t), UV intensity I (z, t) in mW/cm
2; q is the PS triplet state [T] quantum yield; g
= ( Kg k
3) [A]G
o ;
K =1/(1+
C + 0.65[
A]),
G = CYG0 , with
G0 = 1/(Y+
k) k= k5l/+(kil//,[A]. The oxygen supply term is given by
P= POY/fo] ), [O
0]
being the initial oxygen concentration. Parameter (N=10) is added to
fit the measured data of oxygen time- profiles3. s=s1+s2 with s1 and s2
are the fraction of [
3O
2] interacting with [T] to
produce singlet oxygen (in type-II) and other ROS (in type-I),
respectively. In Eq. (2.c), we have defined a new effective
coefficientA’(z,t)=2.3[(a-b)C(z,t)+bC_0+A+Q], a'=ap with p being the
quantum yield for triplet PS state; Q is the tissue absorption
coefficient without nanogold or PS; A is the absorption constant of the
nanogold; a and b are the extinction coefficient of the PS and the
photolysis product having an concentration C(z,t) with initial value C
0.
Eq. (2.b) also includes the light intensity reduction due to the
absorption by nanogold via the exp(-Az) term of Eq. (1.b) when the same
light is used for both PTT and PDT. We note that stronger PTT (or larger
Az) produces higher temperature, which however, also reduces the
available light intensity for PDT. Therefore, there is an optimal
condition depending upon either PTT or PDT will be the dominated process
for optimal clinical outcomes.
Optimal efficacy in PDT
For the PDT dominant case, both type-I and type-II reactions occur in
the photochemical reaction. The kinetic equation of the the
photoinitiation rate for type-I (R
1) process and type-II (R
2)
generation of the reactive oxygen species (ROS) is given by The
anti-cancer efficacy is related to the S-function by Ceff=1-exp(-S),
where S1 (for type-I) and S2 (for type-II) are given by [3]
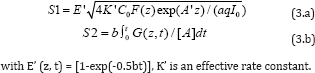
Eq. (2) and (3) can be solved only numerically. For the case for PDT
only, or when PTT is neglected, the Az term in A’ of Eq. (2.c) can be
neglected. We have numerically showed that S1 has an optimal z*, whereas
S2 is a decreasing function of z, and achieves a steady state in time.
It was reported that type-II, or S2, is the predominant process for
anti-cancer, which is governed by the oxygen concentration. S2 reaches a
steady state in time when oxygen is completely depleted.
Optimal synergic effects
As shown by Eq. (3), the S formulas show that
S1 ~ [
I"]05, with no contribution from oxygen [O2]; whereas S2~ [O2]C requires both C and [O2].
Therefore, resupply of PS or oxygen would improve the generation of
ROS, and improve the anti-cancer efficacy via type- II PDT. Figure 3
shows profiles of oxygen and PS concentration, [O2] (red curves] and C (blue curves], for various light intensity of 50, 100, 200 mW/cm2, with external oxygen source P>0, which would improve the type-II efficacy (or S2 function] (Figure 3].
Figure 3: Profiles of oxygen and PS concentration, [OJ (red
curves) and C (blue curves), for various light intensity of 50,100, 200
mW/cm
2 (for curves in dot, solid and dash).
Eq. (2) and (3) show the following important features for the PDT
efficacy defined by the amount of ROS generation. S2 is an increasing
function of the light energy (or intensity x time) and its quantum yield
(q).
However, it is reduced by the PS concentration depletion (with a
quantum yield p). For maximum type-II PDT efficacy one requires the
following conditions: large q (or high quantum yield), sufficient oxygen
supply during the reaction, with P>0), small A (or small absorption
by the nanogold). On the other hand, for high PTT efficacy one requires
large temperature increase (dT) which is proportional to the light
absorption in the nanogold, or AI
0. Therefore, in case-1
using one light to perform both PTT and PDT would require higher light
energy (or intensity) comparing to case-2, using two different lights
for PTT and PDT, independently without co-absorption, and therefore PTT
and PDT can be treated separately. In case-1, the system design is
simpler and cost effective. However, the collective effects between PTT
and PDT require complex optimization of the concentration of PS and
nanogold, besides that the wavelength of the light must match both the
absorption peak of PS and nanogold. Greater details requiring numerical
solutions of Eq. (3) and (5) will be presented elsewhere.
The synergic effects of PTT and PDT, using two lasers at 808 nm and
660 nm, respectively, and nanogold in C6 gel, was reported by Kim et al.
[10]. They reported higher efficacy when conduct PDT prior to PTT than
[PTT+PDT]. This sequential-dependent process may be realized by that PTT
may cause reduction of the kinetic constant and quantum yield due to
increased temperature due to PTT, besides the potential reduction of
oxygen supply which is critical in type-II PDT. In addition to the
methods presented in this paper, the efficacy of PDT may be further
improved significantly via conjugated nanogolds. For example, it was
reported by the conjugated spherical nanogold as the delivery agent for
5-ALA resulted in a two times higher cell death rate compared to free
5-ALA [11]. Another example is that the DNA damage caused by PDT as
demonstrated by alkaline gel electrophoresis was greater in the
methylene blue (MB) plus chitosan-treated group than in control and
MB-treated groups [12-13].
Conclusion
Efficacy of cancer therapy may be enhanced by combining PTT and PDT
either activated by one light or two lights. For maximum PTT/PDT
synergistic efficacy, the concentration of PS and nanogold required
optimization, besides the wavelength of the light matching the
absorption peak of PS and nanogold, and the order of PTT and PDT
process. External supply of either PS or oxygen concentration will
significantly improve the anti-cancer efficacy via type-II PDT, which is
limited by the generation of ROS, or the depletion of oxygen and/or PS
concentration.
Acknowledgment
This work was supported by the internal grant of New Vision Inc. KT
Chen is partially supported by the Ph.D program of Graduate Institute of
Applied Science and Engineering, Fu Jen Catholic University, Taiwan.
Follow on Linkedin : https://www.linkedin.com/company/lupinepublishers
Follow on Twitter : https://twitter.com/lupine_online